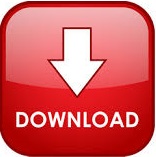
DHFR is an essential enzyme that catalyzes electron transfer reaction to form tetrahydrofolate, a carrier of single-carbon functional groups utilized in specific biochemical reactions ( Harvey and Dev, 1975 Schnell et al., 2004). Here we elucidate a molecular mechanism of GDT by focusing on molecular, systems, and organismal effects of overexpression of E. However, specific mechanisms by which overexpression-induced protein mis-interactions cause toxicity and loss of fitness are not known. Thus, it is plausible that GDT might be caused by protein mis-interactions. Theoretical analyses suggested that the balance between functional and non-functional protein-protein interactions (PPI) is an important determinant of protein abundances in the cell ( Deeds et al., 2007 Zhang et al., 2008 Heo et al., 2011 Wallace and Drummond, 2015). Singh and Dash hypothesized that electrostatic mis-interactions might be responsible for GDT in bacteria, which, unlike yeast, mostly lack proteins with disordered regions ( Singh and Dash, 2013). Vavouri et al hypothesized that GDT in yeast is predominantly caused by disordered proteins because of their potential involvement in multiple protein-protein and protein-DNA interactions, which, under the overexpression regime, could bring about deleterious mis-interactions ( Vavouri et al., 2009).
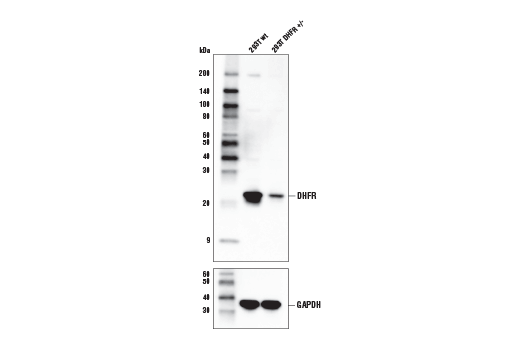
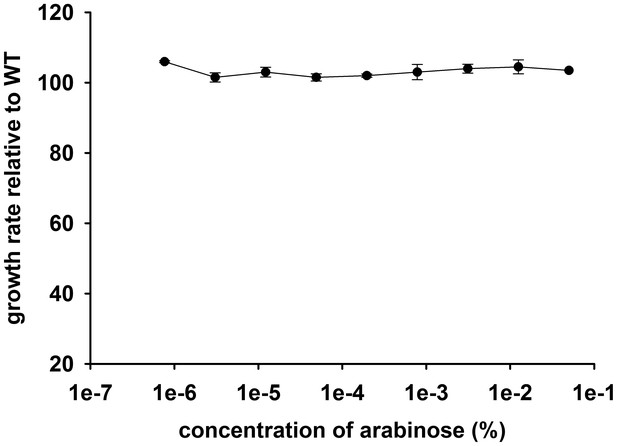
Several mechanisms were proposed to explain gene dosage toxicity (GDT), including resource overload ( Makanae et al., 2013 Shachrai et al., 2010 Snoep et al., 1995 Stoebel et al., 2008), aggregation toxicity ( Geiler-Samerotte et al., 2011 Kaiser et al., 2013), stoichiometric imbalance ( Papp et al., 2003 Veitia et al., 2008), and non-specific PPIs ( Ma et al., 2010 Vavouri et al., 2009). These findings are intriguing, given that gene-dosage increase plays a central role in evolutionary adaptations ( Kondrashov, 2012), such as adaptations to a different carbon source ( Brown et al., 1998), temperature ( Riehle et al., 2001), and emergence of antibiotic resistance ( Andersson and Hughes, 2009). coli, overexpression of the majority of proteins is mildly to severely toxic under the conditions of the experiment ( Kitagawa et al., 2005), whereas in yeast around 15% of overexpressed proteins produce morphological changes and drop in growth ( Sopko et al., 2006). High-throughput studies revealed that overexpression of a substantial fraction of genes is detrimental to fitness. Phenotypes produced by gene overexpression generate a plethora of fitness effects that tend to deviate from those observed in gene deletion studies ( Sopko et al., 2006 Prelich, 2012). This study shows how protein overexpression perturbs a dynamic metabolon of weak yet potentially functional PPI, with consequences for the metabolic state of cells and their fitness.Įxperimental approaches to mapping the functional relationships between genes and phenotypes traditionally use perturbations of gene dosage via systematic deletion ( Baba et al., 2006 Giaever et al., 2002 Pan et al., 2004 Sopko et al., 2006), down-regulation ( Mnaimneh et al., 2004), or overexpression ( Sopko et al., 2006 Gelperin et al., 2005 Kitagawa et al., 2005 Makanae et al., 2013) of the target genes. coli’s self’ and ‘foreign’ proteins suggests the crucial role of evolutionary selection in shaping protein-protein interaction (PPI) networks at the whole proteome level.
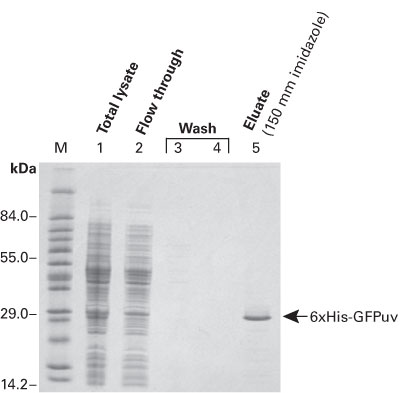
Moreover, we found that overexpression of orthologous DHFR proteins had minimal effect on all levels of cellular organization – molecular, systems, and phenotypic, in sharp contrast to E. Though deleterious in the overexpression regime, surprisingly, these interactions are beneficial at physiological concentrations, implying their functional significance in vivo. coli causes a toxic metabolic imbalance triggered by interactions with several functionally related enzymes. Here, we demonstrate that overexpression of DHFR in E. Gene dosage toxicity (GDT) is an important factor that determines optimal levels of protein abundances, yet its molecular underpinnings remain unknown.
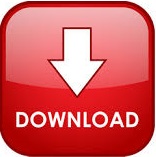